What does intense internal heat signify, and how does it impact various processes? A deep understanding of extreme thermal conditions is crucial for numerous applications.
The term describes a high level of internal temperature. This extreme heat can manifest in various contexts, such as within the Earth's core, during the operation of specialized industrial equipment, or within specific physiological conditions. Examples include the intense heat generated during a nuclear reaction or the scorching temperatures in a forge. Understanding the properties of materials at these extreme temperatures is paramount for designing and maintaining equipment able to withstand such conditions, and has vital implications for materials science and engineering.
The importance of understanding and managing extreme heat lies in the potential for both beneficial and detrimental consequences. Extreme heat, in specific controlled settings, is essential to several industrial processes, including smelting, forging, and manufacturing specific materials with unique properties. Moreover, comprehending the behavior of materials under high internal temperature is fundamental in geophysics, astrophysics, and numerous other scientific disciplines, allowing scientists to model geological formations and stellar evolution. The historical context of studying extreme heat demonstrates humanity's consistent need for innovative solutions to harness and control these phenomenal conditions.
This exploration of intense internal heat transitions to further discussions on thermal management in various scientific and industrial contexts. Understanding extreme temperature environments is pivotal to developing sustainable and efficient solutions for critical applications. This will be followed by in-depth analysis of specific instances and their implications.
Deep Hot
Understanding the multifaceted nature of "deep hot" requires a nuanced approach. The following aspects illuminate crucial dimensions of this complex concept.
- Extreme temperature
- Internal heat
- Material properties
- Industrial processes
- Geological phenomena
- Energy generation
- Thermal management
- Scientific research
These aspects, individually and in concert, contribute to the comprehensive understanding of "deep hot." Extreme temperature, for instance, directly influences material properties, as seen in the behavior of metals during forging. Internal heat, like that within the Earth's core, drives geological phenomena. Industrial processes, such as smelting, rely on controlled deep hot conditions. Understanding these aspects collectively fosters innovations in energy generation and thermal management strategies, ultimately informing scientific research and technological advancements.
1. Extreme Temperature
Extreme temperature, a defining characteristic of "deep hot" conditions, plays a critical role in shaping the behavior of materials and driving various processes. The intensity of this heat influences properties such as strength, ductility, and reaction rates, profoundly impacting applications ranging from industrial manufacturing to geological formations.
- Material Degradation and Enhancement
Extreme temperatures significantly alter material properties. Metals, for instance, become more brittle at high temperatures, impacting their structural integrity. Conversely, specific heat treatments exploit these temperature-dependent changes to enhance material strength or create unique functionalities. This interplay between extreme heat and material properties is crucial in industrial processes requiring high-temperature operation.
- Driving Geological Processes
Elevated temperatures within the Earth's interior are fundamental drivers of geological processes. Magma formation, volcanic activity, and plate tectonics are examples of phenomena directly linked to the extreme heat within the Earth. Understanding these processes requires analyzing the effects of extreme temperature on the composition and behavior of Earth's materials.
- Industrial Applications and Constraints
Many industrial processes, such as refining, forging, and manufacturing certain alloys, operate at extreme temperatures. This necessitates specialized equipment capable of withstanding these conditions. Material selection, design considerations, and process optimization are crucial to ensure safe and efficient operation within these high-temperature environments.
- Impact on Chemical Reactions
Extreme temperatures catalyze chemical reactions. These accelerated reactions are vital in various industrial applications, influencing the efficiency of chemical synthesis, refining processes, and specific high-temperature catalytic conversions. Understanding the influence of extreme heat on reaction kinetics and rates is essential for optimizing chemical processes.
In summary, extreme temperature is not merely a characteristic of "deep hot" but a driving force behind phenomena and processes across diverse disciplines. Understanding the complex interplay between temperature and material properties is paramount for advancing industrial technologies, interpreting geological activities, and optimizing chemical reactions.
2. Internal Heat
Internal heat, a defining component of "deep hot" conditions, signifies the thermal energy residing within a system or substance. Its intensity and distribution are crucial determinants of the characteristics and consequences associated with these conditions. Internal heat, as a driving force, influences material behavior, process outcomes, and observable phenomena in diverse contexts. In geological formations, internal heat powers processes like magma generation and volcanic activity. In industrial settings, internal heat is harnessed in various processes, including forging and smelting. The profound impact of this internal energy underscores its importance within the broader scope of "deep hot."
The significance of internal heat as a component of "deep hot" extends to numerous practical applications. The controlled generation and management of internal heat are vital in industrial processes, from manufacturing alloys to refining metals. In these settings, precise control over temperature gradients and heat distribution is essential for achieving desired outcomes. The ability to understand and predict the effects of internal heat on material properties is crucial for optimizing material selection, equipment design, and process efficiency. Likewise, the understanding of internal heat flow in geological systems is fundamental for predicting and mitigating the hazards of volcanic activity and earthquakes. Accurate models of internal heat distribution enable improved resource management and environmental monitoring in these settings.
In conclusion, internal heat is an essential component of "deep hot" conditions. Its influence extends beyond the realm of theoretical concepts to practical applications in numerous scientific and industrial domains. Precise control and management of this internal energy are crucial for achieving desired outcomes. Understanding its underlying principles and effects across various systems remains a vital area of scientific inquiry, directly impacting both technological advancements and hazard mitigation.
3. Material Properties
Material properties exhibit a profound dependence on extreme temperature conditions. "Deep hot" environments significantly alter material characteristics. This alteration is not merely a theoretical concept; it has real-world implications in diverse fields, from metallurgy to geophysics. Elevated temperatures impact fundamental properties like strength, ductility, and thermal conductivity. These changes can be beneficial or detrimental, depending on the specific application and the desired outcome.
Consider the behavior of metals under intense heat. At elevated temperatures, many metals experience a reduction in tensile strength and ductility. This makes them more susceptible to fracture and deformation. Conversely, specific heat treatments, precisely controlling temperature, can enhance strength and hardness. The forging of steel, for instance, relies on this principle: manipulating the material's microstructure under high temperatures to achieve a desired level of strength. Similarly, understanding how different materials react at high temperatures is vital for designing components in extreme environments, such as high-temperature turbines in jet engines or heat exchangers in nuclear power plants. Failure to accurately predict material behavior under deep hot conditions can lead to catastrophic failures with significant safety and economic consequences. In geophysics, the behavior of rocks and minerals at high pressures and temperatures dictates the Earth's geological processes, from mountain formation to volcanic eruptions.
The interplay between material properties and "deep hot" conditions is multifaceted and crucial for advancements in diverse fields. A thorough understanding of how materials respond to extreme heat allows for the development of novel materials resistant to degradation at high temperatures. This has applications in enhanced energy production, advanced manufacturing techniques, and exploration of extreme environments. Challenges remain in predicting material behavior under highly complex and often unpredictable "deep hot" conditions, necessitating further research and development.
4. Industrial Processes
Industrial processes frequently necessitate the creation and management of "deep hot" conditions. This necessitates a profound understanding of material behavior under extreme temperatures and pressures. Optimization of these processes hinges on precise control of thermal parameters, impacting efficiency, safety, and cost-effectiveness.
- Smelting and Refining
Many metallurgical processes, including smelting and refining, operate at elevated temperatures. These processes involve extracting metals from ores or purifying existing metals. High temperatures are crucial for initiating chemical reactions, enabling the separation of desired elements, and achieving the desired purity levels. Inadequate control of temperature gradients can result in inferior metal quality, production inefficiencies, or safety hazards.
- Forging and Heat Treatment
Forging and heat treatment processes rely on controlled high temperatures to alter the microstructure and mechanical properties of metals. Specific heating and cooling cycles, precisely calibrated, produce desired levels of hardness, toughness, and strength in metals. Precise control over the temperature profile during forging and heat treatments is essential for producing components with the requisite properties for the intended application.
- Glass Manufacturing
Glass production requires high temperatures to soften and shape the raw materials. The precise temperature control dictates the final characteristics of the glass, such as its viscosity, transparency, and durability. Understanding how temperature affects glass formation is crucial for achieving the desired product quality and maintaining production efficiency.
- High-Temperature Chemical Reactions
Certain chemical processes are significantly influenced by elevated temperatures. These processes are vital in industrial settings, particularly in refining, petrochemical production, and manufacturing specialized materials. Controlled high temperatures can accelerate chemical reactions, increasing production rates. Failure to maintain optimal temperature profiles can lead to undesired byproducts, decreased yield, or equipment damage.
In summary, industrial processes frequently leverage "deep hot" conditions for various transformations and material modifications. The efficient and safe operation of these processes hinges on precise control and understanding of the intricate interplay between heat, materials, and equipment. This requires thorough knowledge of material behavior at high temperatures, accurate process monitoring, and specialized equipment capable of withstanding these extreme conditions.
5. Geological Phenomena
Geological phenomena are inextricably linked to "deep hot" conditions. The Earth's internal heat engine, operating at immense temperatures and pressures, directly drives a multitude of geological processes. Understanding these interactions is fundamental to comprehending the Earth's dynamic systems and their influence on the planet's surface.
- Magma Generation and Volcanic Activity
High internal temperatures within the Earth's mantle facilitate the melting of rock, generating magma. This molten rock, driven by buoyancy forces, ascends toward the surface, leading to volcanic eruptions. The intensity and frequency of volcanic activity are directly correlated with the extent and distribution of deep heat within the Earth's interior. Understanding these relationships is crucial for predicting volcanic hazards and assessing the geological risks associated with volcanic regions.
- Plate Tectonics
Convective currents within the mantle, driven by heat differences, are a primary force behind plate tectonics. The movement of tectonic plates is a consequence of the Earth's internal heat engine. This movement is responsible for earthquakes, mountain building, and the formation of oceanic trenches. The interplay between deep heat and plate motion shapes the Earth's surface over vast geological timescales.
- Metamorphism
Extreme temperature and pressure conditions deep within the Earth lead to the transformation of existing rocks into metamorphic rocks. This process alters the mineralogy, texture, and structural properties of rocks. The specific metamorphic processes are directly influenced by the temperature and pressure gradients resulting from the deep heat. This understanding is crucial in interpreting the geological history of regions and identifying subsurface features.
- Formation of Ore Deposits
Deep-seated heat promotes the chemical reactions and transport processes that contribute to the formation of ore deposits. The hydrothermal solutions circulating at depth, heated by the Earth's internal heat, carry dissolved metals and minerals. These solutions are vital in creating economically significant ore deposits. The study of these processes provides insights into the spatial and temporal distribution of mineral resources.
In conclusion, the profound influence of "deep hot" conditions on Earth's geological phenomena is undeniable. From the molten heart of the planet to the surface manifestation of volcanic activity and the slow but relentless shift of tectonic plates, internal heat is the driving force behind many geological processes. Thorough understanding of these processes is vital for assessing natural hazards, comprehending the Earth's history, and developing strategies for sustainable resource management.
6. Energy Generation
Energy generation frequently leverages "deep hot" conditions, particularly in nuclear power and geothermal energy production. These applications exploit the immense energy reserves locked within the Earth's interior or harnessed from controlled nuclear reactions, highlighting a crucial link between high temperatures and energy production. The feasibility and safety of these methods demand a thorough understanding of material behavior, thermal management, and the associated risks.
- Nuclear Power and Controlled Nuclear Fission
Nuclear power plants utilize controlled nuclear fission to generate heat. This heat is then used to produce steam, which drives turbines to generate electricity. The process inherently involves extremely high temperatures and pressures, demanding specialized materials capable of withstanding these conditions. Safety protocols and material science advancements are paramount to mitigate risks associated with these high-energy environments.
- Geothermal Energy and Heat Flow
Geothermal power plants tap into the Earth's internal heat. The heat is extracted from deep underground reservoirs, either through direct steam extraction or by circulating water heated by the Earth's mantle. This method relies on the Earth's naturally occurring heat flow, demonstrating the inherent energy reserve in "deep hot" conditions. Careful consideration of geological formations, water quality, and potential environmental impacts is essential.
- Material Science and High-Temperature Tolerance
The success of energy generation methods relying on "deep hot" conditions depends critically on materials science. Developing materials that can withstand extreme temperatures and pressures is vital for reactor components, pipelines, and other crucial elements. Research focuses on materials' mechanical properties, thermal conductivity, and their ability to resist corrosion and degradation at high temperatures and pressures.
- Safety Regulations and Risk Mitigation
Maintaining safety and mitigating risks are paramount in energy generation methods using "deep hot" conditions. Rigorous safety regulations, comprehensive risk assessments, and robust containment systems are essential for preventing accidents and ensuring public safety. Continuous monitoring and maintenance procedures are critical components in managing potential hazards associated with the extreme thermal conditions.
In conclusion, energy generation frequently depends on harnessing the immense energy available in "deep hot" environments. From nuclear fission to geothermal energy, these approaches capitalize on the Earth's internal heat or controlled nuclear reactions. This dependence underscores the crucial role of advanced materials science, effective thermal management, and meticulous safety protocols in guaranteeing the safe and efficient generation of energy using these extreme conditions.
7. Thermal Management
Thermal management is critical in applications involving "deep hot" conditions. Maintaining precise temperature control is paramount for safety, efficiency, and the successful operation of various systems. Effective thermal management strategies mitigate potential damage and ensure reliable performance under extreme thermal environments. This involves careful consideration of heat transfer mechanisms, material properties, and system design.
- Heat Transfer Mechanisms
Understanding heat transfer mechanisms, including conduction, convection, and radiation, is fundamental in thermal management strategies for "deep hot" environments. Each mechanism operates differently and plays a distinct role in the overall thermal behavior of a system. In extreme heat applications, minimizing heat loss or efficiently distributing heat is key. For instance, in nuclear reactors, heat generated from nuclear reactions must be effectively transferred to external systems without compromising containment or safety.
- Material Selection and Properties
Material selection for components operating in "deep hot" environments is critical. Materials must possess high thermal stability, resistance to degradation at elevated temperatures, and appropriate thermal conductivity. Different materials exhibit varying performance characteristics under extreme heat, and choosing the right material for a specific application significantly impacts the system's overall thermal management. High-temperature alloys are essential in applications requiring robust structural integrity under intense heat.
- System Design and Configuration
System design plays a vital role in effective thermal management. Factors such as geometry, insulation, and fluid flow patterns must be carefully considered. Specific designs optimize heat transfer pathways, enhance thermal stability, and prevent thermal shocks or runaway temperatures. The configuration of heat exchangers, for instance, is a critical design aspect in numerous thermal management applications, especially those involving "deep hot" conditions.
- Monitoring and Control Systems
Sophisticated monitoring and control systems are indispensable for managing complex thermal systems under "deep hot" conditions. Real-time temperature monitoring, coupled with automated control mechanisms, allows for precise adjustments to maintain optimal operating temperatures and prevent thermal anomalies. Advanced sensors and control systems ensure safety and optimize performance, mitigating risks associated with uncontrolled heat buildup in high-temperature environments.
In conclusion, effective thermal management in "deep hot" environments is a multi-faceted approach. It necessitates a deep understanding of heat transfer principles, suitable material selection, meticulous system design, and robust monitoring and control systems. Implementing these strategies leads to safer, more efficient, and reliable operation of systems subjected to intense thermal conditions. This approach is crucial for various industrial applications, including metallurgy, energy generation, and scientific research, where precisely controlled thermal conditions are vital.
8. Scientific Research
Scientific research plays a critical role in understanding and mitigating the challenges posed by "deep hot" conditions. Investigations into extreme temperatures and associated phenomena are vital for developing materials, technologies, and strategies for managing these conditions safely and effectively. Research encompasses a broad spectrum, from fundamental studies of material behavior at high temperatures to applied investigations of industrial processes operating under extreme thermal constraints. This exploration necessitates a meticulous approach encompassing diverse scientific disciplines.
- Material Science and High-Temperature Behavior
Research into material science is essential for developing materials resistant to degradation and failure under "deep hot" conditions. Investigations focus on the mechanical properties, thermal conductivity, and chemical stability of various substances at extreme temperatures. Studies analyze phase transitions, microstructure changes, and the evolution of material properties under sustained high heat. This research has implications for designing components in high-temperature applications, such as advanced engines, nuclear reactors, and aerospace technologies.
- Geophysical Investigations of the Earth's Interior
Understanding the Earth's internal heat flow and its impact on geological processes is crucial. Research explores the mechanisms driving magma generation, plate tectonics, and volcanic activity, often employing advanced modeling and geophysical techniques. Insights gained from these studies contribute to the prediction and mitigation of natural hazards associated with extreme thermal conditions within the Earth.
- Chemical Kinetics and Reaction Mechanisms at High Temperatures
Research into chemical reactions at elevated temperatures provides a deeper understanding of their kinetics and mechanisms. Studies analyze how reaction rates, equilibrium constants, and product distributions vary with temperature, including the design of more efficient industrial processes operating under high-temperature conditions. This information is vital for optimizing processes in refining, manufacturing, and chemical synthesis.
- Thermodynamic Analysis of Extreme Temperature Systems
Thermodynamic models are critical for comprehending the intricate relationships between heat, work, and energy in high-temperature systems. Research focuses on accurately modeling energy transfer, heat capacity, and entropy variations under extreme conditions. These models are foundational for designing efficient thermal management strategies in diverse applications, including energy production and manufacturing.
In conclusion, scientific research underpins the understanding and management of "deep hot" conditions. Through rigorous investigations into materials science, geophysics, chemical kinetics, and thermodynamics, researchers continually unveil new knowledge. This understanding is vital for innovation in various technological domains and for mitigating risks associated with the Earth's dynamic processes.
Frequently Asked Questions about "Deep Hot"
This section addresses common inquiries related to "deep hot" conditions, encompassing various scientific and industrial applications. Questions and answers are presented in a straightforward and informative manner.
Question 1: What are the key characteristics defining "deep hot" conditions?
Answer: "Deep hot" conditions are characterized by exceptionally high internal temperatures, often accompanied by significant pressures. These conditions can manifest in diverse contexts, including the Earth's interior, industrial processes, and controlled laboratory environments. The precise definition and parameters depend on the specific application.
Question 2: How do material properties change under "deep hot" conditions?
Answer: Material properties, such as strength, ductility, and thermal conductivity, undergo substantial transformations at elevated temperatures associated with "deep hot" conditions. These changes can be detrimental or beneficial, depending on the intended application. For example, certain metals become more brittle at high temperatures, necessitating careful material selection and processing considerations.
Question 3: What are some examples of industrial processes utilizing "deep hot" conditions?
Answer: Numerous industrial processes rely on "deep hot" conditions. Smelting, forging, and certain chemical reactions are examples where extreme temperatures are integral to the process. The manipulation of materials at these temperatures facilitates desired structural transformations, chemical reactions, and product formation.
Question 4: How do "deep hot" conditions influence geological phenomena?
Answer: The Earth's internal heat, a form of "deep hot" condition, directly influences geological processes. Magma formation, volcanic activity, and plate tectonics are examples of processes driven by these extreme temperatures and pressures. Understanding these interactions is critical for assessing geological risks and resource potential.
Question 5: What role does scientific research play in managing "deep hot" conditions?
Answer: Scientific research is indispensable for developing strategies to manage and utilize "deep hot" conditions. Research focuses on materials science, geophysics, and thermodynamics, enabling improved material development, more efficient industrial processes, and safer operations under extreme temperatures. This knowledge significantly reduces risks and facilitates the responsible use of these powerful conditions.
In summary, "deep hot" encompasses a spectrum of conditions characterized by intense heat. Understanding the material behavior, geological implications, and industrial applications associated with these conditions is crucial for safe and efficient practices across diverse disciplines.
The subsequent section will delve into specific applications of "deep hot" conditions, further illustrating their multifaceted relevance in various contexts.
Conclusion
This exploration of "deep hot" conditions reveals a multifaceted phenomenon impacting diverse fields. The extreme temperatures associated with these conditions profoundly alter material properties, driving industrial processes, influencing geological phenomena, and playing a critical role in energy generation. Key findings highlight the necessity for meticulous thermal management strategies, advanced materials science, and robust safety protocols to mitigate risks associated with these intense environments. The interplay between heat, material behavior, and system design dictates the success and safety of applications ranging from metallurgy to geophysics. The scientific understanding and technological advancements concerning "deep hot" conditions are not merely academic pursuits but critical for responsible resource management and mitigating potential hazards.
Moving forward, research must continue to address the complexities of "deep hot" phenomena. Further investigation into material behavior under extreme conditions, coupled with innovative thermal management strategies, promises to unlock new possibilities for industrial advancement and a deeper understanding of the Earth's dynamic systems. This knowledge, underpinned by rigorous scientific inquiry, is essential for harnessing the potential of these extreme environments while ensuring safety and sustainability for future generations.
You Might Also Like
Challenging Taboos: Pushing Boundaries & FrontiersVika And Vova: Viral Video Highlights!
New MMS India: Latest Updates & Services
Sophie Raid: Latest News & Updates
Charming Czech Streets: Explore The Best!
Article Recommendations
- Hank 3 The Multifaceted Musician And His Impact
- How Many Times Has Damon Imani Been On The View
- Jesse Plemons A Rising Star In Hollywood
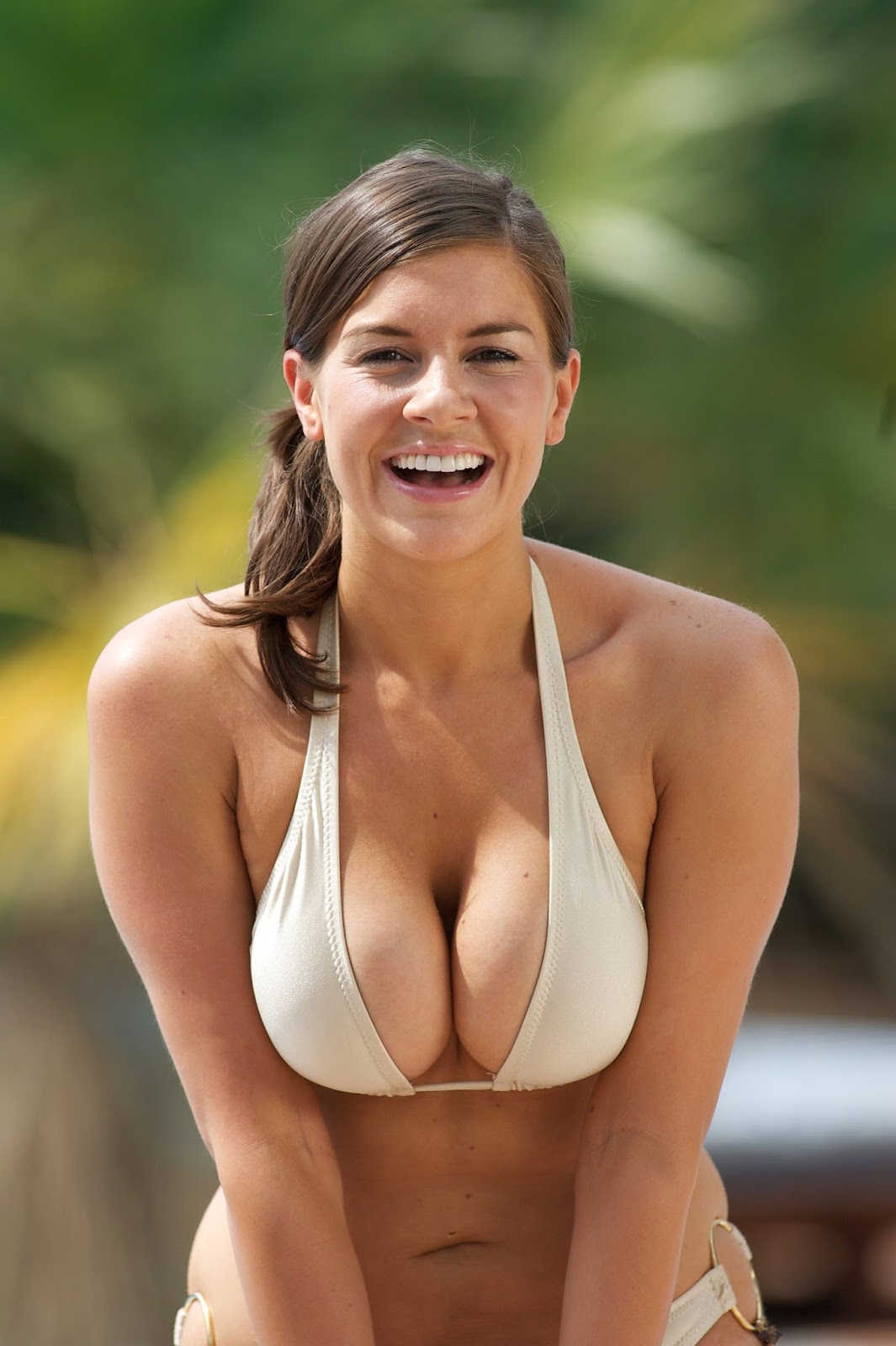

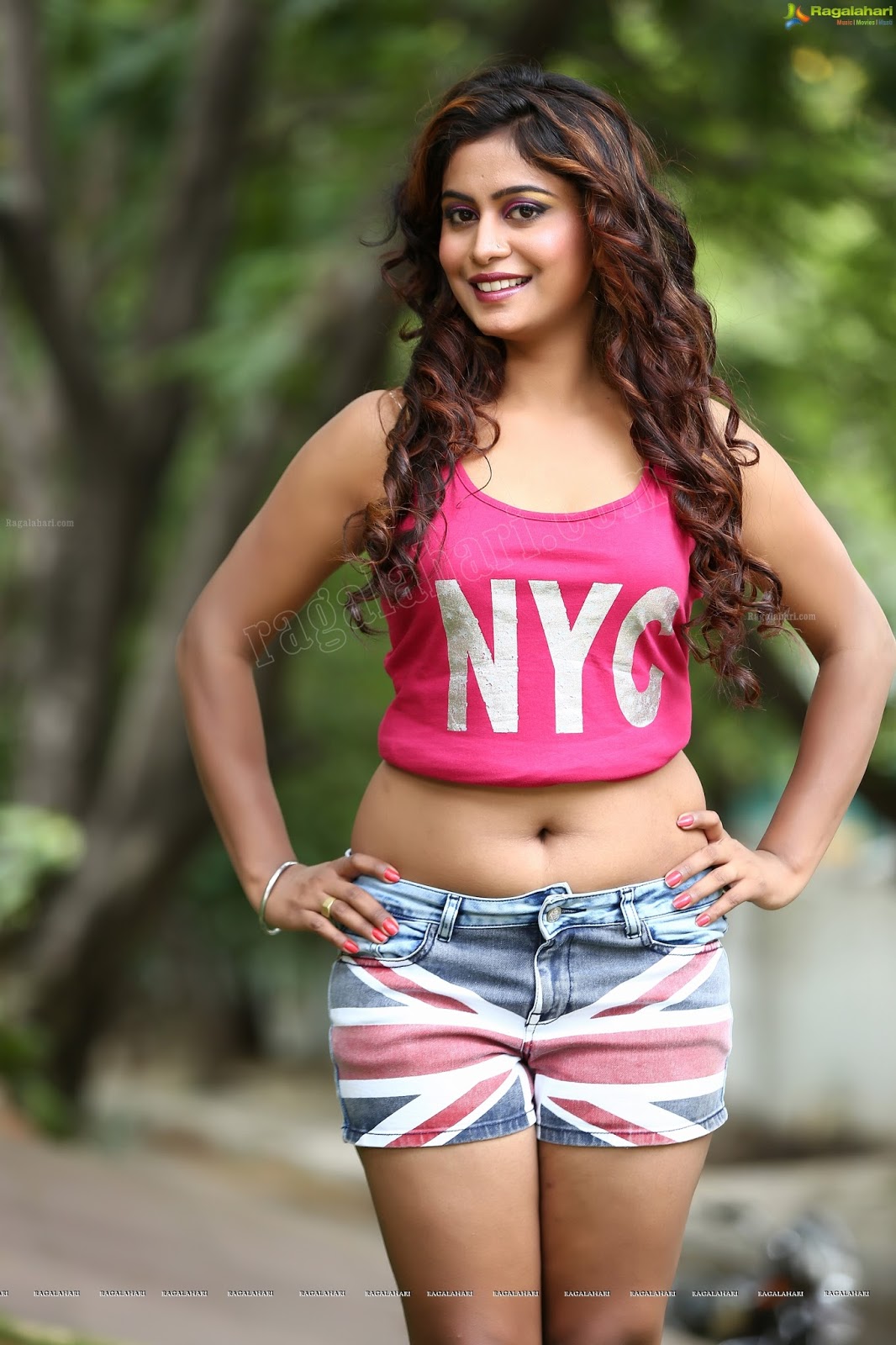